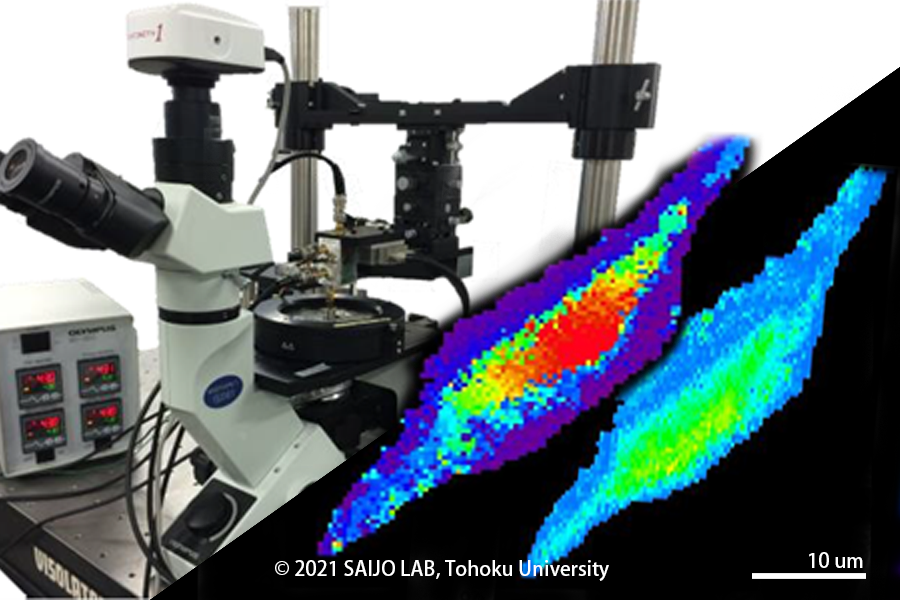
Acoustic Microscope
Non-invasive imaging of microstructures inside cell and on the surface of the skin
The spatial resolution of an ultrasound image increases in proportion to the freqeuncy of the ultraosund waves. Conventional frequencies used in medical ultrasound imaging range from 2 to 15. MHz, but in our laboratory, we use to 300 Mhz We are developing systems and signal processing technologies for "acoustic microscopes," which can visualize the microstructure of an object on a micron scale using a MHz ultrasonic transducer.
Unlike ordinary optical microscope, ultrasound microscopes can visualize the three-dimensional distribution of mechanical properties such as hardness and viscoelasticity of tissue sections without staining, and are expected to be used in a wide range of healthcare fields such as tissue diagnosis of tumors, diagnosis of srterial plaque, and measurement of skin moisture content.
Ultrasound frequency and spatial resolution
Ultrasound is well known as a safe and portable diagnostic device, but from an engineering poin of view, it is more than just these "conveniences"; it is modality with high spatial and temporal resolution. Since the spatial resolution is inversely proportional to the frequency, the use of high-frequency ultraosund enable high-resolution tissue imaging. The figure on the left shows the relationship between frequency and spatial resolution of ultrasound currently used in clinical appliications. Clinical applications include abdominal echocardiography and echocardiography in the freqency range of several MHz, carotid artery echocardiography and echocardiography of body surface tissues such as thyroid, breast, and muscle in the frequency range of more than ten MHz, and intravascular ultrasound in the frequency range of 20-45 MHz. Ultrasound microscopy uses ultrasound waves with a frequency of 100 MHz or higher to achieve imaging with a resolution of about 15 microns (roughly equivalent to 40 times that of an optical microscope). Ultrasound at higher frequencies (e.g., in the GHz range) provides a resolution of about 1 micron, which is enough to observe a single cell.
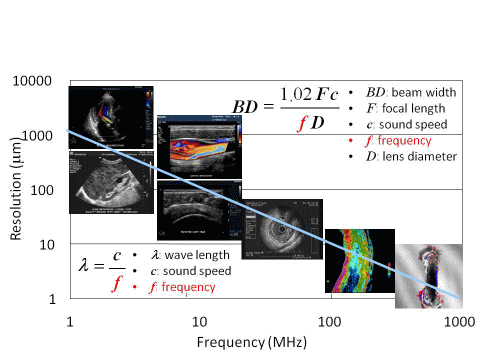
Principle Acoustic Microscope
In an acoustic microscope, the plane wave emitted from a plane transducer is focused by an aocustic lens such as sapphire or by a concave transducer to narrow down the ultrasonic wave to almost the same level as the wavelength of the focus. Currently mechanical scanning ultrasound microscope are the mainstream, in which a transducer scans a tissue section or cultured cells on a glass slide in two dimensions to obtain an image. The tissue does not need to be specifically stained, and the scan takes about 10 seconds to complete if it is just imaging, or abut a minute if it is acquiring acoustic data. Since 2001, we have been working on digitization of the ultrasonic microscope, and now all the reflection waveforms of each point are stored as digital data, which enables quantitative analysis of various acoustic parameters such as sound velocity, attenuation, and acoustic impedance.
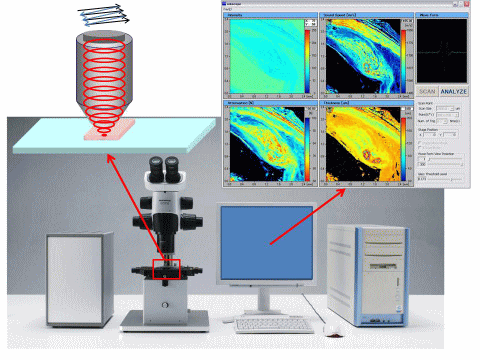
What can we learn from acoustic microscopy?
Our research group started the medical application of acoustic microscopy in 1985. Originally, we started our reserach with the aim of being able to observe tissues without staining during rapid intraoperative diagnosis, but out research gradually evolved to the purpose of investigating the source of echoes in clinical ultraosund images. Recently, as shown in the equation on the right, we have also been using acoustic parameters to measure the elasticity and viscosity of tissues at the microscope level for the purpose of exploring the biomechanics of biological tissues. I have been conducting research on organs such as the heart and atherosclerosis, where tissue biomechanics has a significant impact on the pathology, and have recently expanded my research to include orthopedics and dentistry, where biomechanics is also important.
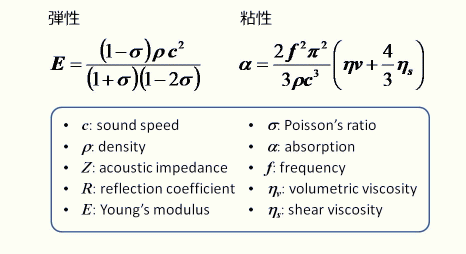
Application of Acoustic Microscopy
The figures below show ligh and ultrasound microscopy (attenuation and sound velocity) images of myocardial infarction (top) and atherosclerosis (bottom). The collagen fibers that develop after myocardial infarction have long been thought to be the source of strong echogenicity in myocardium, and in fact, sound velocity images showed that the speed of sound was faster in the collagen region. In fact, the spped of sound images showed that the collagen region had a higher speed of sound. However, when considering it as a source of echoes, it was necessary to take into account the difference in acoustic impedance with the surrounding tissue. It was also found that the speed of sound slows down in chronic diseases such as dilated cardiomyopathy even in the same collagen fibers. Atherosclerosis tissue is characterized by thickened intimal fibrosis and fat accumulation in the intima, but acoustic microscopy has shown that fibrotic tissue has a high speed of sound and fatty tissue has a low speed of sound. In terns of the relatioship between acoustic parameters and tissue elasticity, the soft adipose tissue is covered by the relatively hard fabrotic tissue. Finite element analysis based on these measuremennts revealed that tissue stress concentrates in these areas and leads to plaque failure.
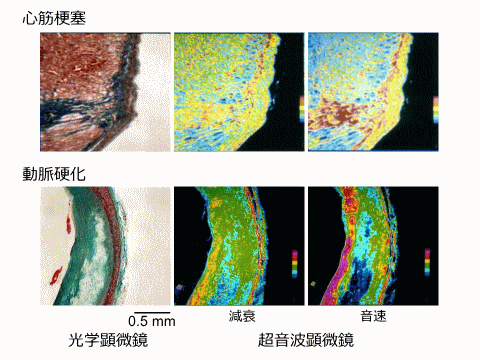
Ultrasonic Impedance microscope
Although ultrasound microscopy was successful in acoustically coloring unstained tissues, it required special equipment and efforts similar to that required to prepare specimens for light microscopy, becuase it required cutting the target into thin slices. The ultrasound impedance microscope was developed in collaboration with Professor Naohiro Hozumi of Aichi Institute of Technology. Conventional ultraosund microscopes capture ultraosund waves transmitted through tissue and reflected on a glass surface, but the ultrasound impedence microscope caotures ultraosund waves transmitted through a plastic plate and reflected on the tissue surface. Since biological tissue is soft, its boundary surface becomes almost flat when it adheres to the plastic plate, and by scanning this boundary surface, we can obtain an image of acoustic impedance distribution on the tissue surface. Therefore, it is no longer necessary to cut the tissue into thin slices, and the tissue can be easily observed. The figure on the right is a cross-section of a rat cerebellum, obtained in collaboration with Dr. Shoko Yoshida of Toyohashi University of Technology. Recently, ultraosund impedance microscopy has also been applied to an international collaborative study on how wrinkles form in the brain.
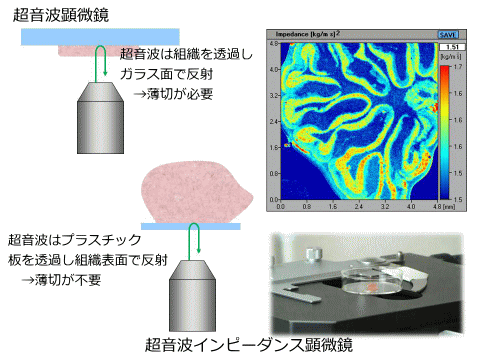
Skin Imaging
The development of ultrasonic microscopes using a series of new technologies, such as ultrasonic impeddance micrpscopy and ultrasonic sound velocity microscopy, has led to the development of three-dimensional ultraonic microscopes that can directly observe the three-dimensional structure of biological tissues. The figure on the right shows a cross-sectioiinal image perpendicular to the skin surface and a cross-sectional images parallel to the skin surface using a three-dimensional ultrasonic micrscope. It is now possible to observe so-called skin texture, microvessles in the dermis, and hair follicles. In April 2010, I became a member of the Smart Aging International Research Center and started comprehensive research on skin imaging amomg three major aging processess for modern people: brain, hair, and skin. It was found that the size and number of collagen and sebaceous glands in the dermis are strongly related to skin elasticity and sebaceous gland volume.
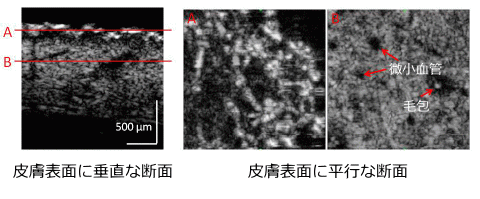